Acoustics of the yidaki or didjeridu
This page is an appendix to two scientific papers, one
experimental and one
theoretical about the yidaki or didjeridu (didgeridoo) and
its interaction with the vocal tract . It presents sound files,
videos and spectra. It also shows some measurements of the acoustical
impedance spectrum of the player's vocal tract, measured just
inside the lips, while he was playing.
There is also an introductory page of Acoustics
of the Yidaki/ Dijeridu, which has a wider range of sounds.
Lip motion
When playing the yidaki, the lips move much like those of a
tuba player. The image and video clip below were taken with
a camera operating at 1000 frames per second. The "yidaki" in
question was made with glass panels. A mirror is positioned
so that, while the main image is from the front, a side view
of the lips is shown at right. The scales on the left and right
are in millimetres.
The same transparent "yidaki" was also used to make schlieren
images of the air jet from the lips. These are shown in the
animation below and in Figure 8 of the experimental
paper.) Click on the image for video clip.
Circular breathing
One of the features of yidaki playing is the use of "circular
breathing". In the sound file below, the player begins with
a steady, sustained note. This is followed by a series of alternations
between normal playing and inhalations. During the inhalations,
which are made through the nose, the player continuges to play
using air stored in the mouth cavity, which is isolated from
the nasal cavity by the soft palate. The air is expelled from
the mouth using tension in the muscles of the distended cheeks.
The part of the sound file with the three circular breaths is
analysed in Figure 10 of the experimental
paper.)
. . . Download sound file in .wav format (400 k)
Vocal tract resonances
The differences in sound between the inhalation and normal playing
phases are due, in part, to the difference between the resonances
in the player's mouth alone (inhalation phase) and in the vocal
tract (normal playing).
Players can also make spectacular changes in the spectral
envelope of the sound produced using the position of the tongue
to change the frequency response of the vocal tract. In particular,
positioning the tongue close to the hard palate (the "high
tongue" position) produces a strong formant between 1 and
2 kHz, its exact value depending on details of the mouth geometry.
With the tongue low in the mouth, this formant disappears.
The next sound file consists of a sample of the "high tongue"
sound, followed by a sample the "low tongue" sound. This pair
of samples is repeated three times. Spectra of the two sounds
are shown below.
. . . Download sound file in .wav format (700 k)
As we explain in the papers mentioned above, the spectral envelope
of the sound produced when playing the yidaki depends strongly
on the resonances of the player's vocal tract. A maximum in
the acoustic impedance of the tract at a particular range of
frequencies inhibits flow into the instrument at those frequencies
and thus produces a broad minimum in the spectral envelope.
Conversely, a minimum in the impedance of the tract produces
a formant or enhanced band of frequencies in the output sound.
To produce the "high tongue" or high
drone sound, the tongue is held close to the hard palate
to make a narrow constriction. Consequently, the acoustic
impedance has high values at the resonances, as shown
in the figure at left below. The frequencies at which
the impedance is high correspond to resonances that have
pressure antinodes and velocity nodes near the lips. Consequently,
there is very little acoustic flow into the instrument
at these frequencies, and so a minimum in the spectral
envelope (figure at left). Between these minima, the flow
is not impeded so much, and so there are formants, or
peaks in the spectral envelope, at frequencies at which
the impedance is low. |
To produce the "low tongue" sound,
the tongue lies low in the mouth (figure at right). Even
at the resonant frequencies, the acoustic impedance is
lower, because of the larger aperture. Consequently, the
acoustical flow at these frequencies is not inhibited
substantially and there are no strong formants. Note the
absence of the strong formant in both the spectrum and
the sound files. Note that, in the sound files, the pitch
as well as the timbre is changed by the tongue position.
We have reported on this effect in the trombone in another
study.
|
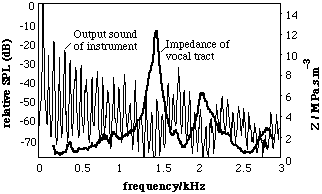
Sound spectrum and vocal tract impedance
for "high tongue" configuration |
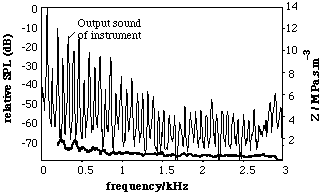
Sound spectrum and vocal tract impedance
for "low tongue" configuration |
The curves are typical. In the high tongue configuration (left),
maxima in of the spectrum of the output sound correlated well
with minima in the impedance spectrum (a correlation coefficient
0.98 for 46 measurements on three players).
In the sound file, although the sound is clearly that of
a yidaki with a pitch below that of the normal human voice.
Nevertheless, it sounds a little like someone alternating
between the sound "ee" (the vowel in the English word "heed")
and an indistinct vowel something like "aw" (the vowel in
"hoard" or "hot"). In a previous study, we measured the resonances
of the vocal tracts during speech. Vocal tracts pronouncing
"heed" have a strong resonance at about 1.8 kHz, while tracts
pronouncing "hoard" or "hot" have no resonances between 1
and 2 kHz.
In our papers on the yidaki, we briefly discuss the importance
of the glottis (the aperture left open between the vocal folds)
in the production of strong resonances in the vocal tract
in the kHz region. When the vocal folds are nearly closed,
as they are for speech, the reflection coefficient for sound
waves travelling down the vocal tract is high for all but
very low frequencies. When the vocal folds are relaxed and
open, the reflection coefficient for frequencies near 1 kHz
is much lower: sound waves in the upper airway are more readily
transmitted to the lower airway and to the highly lossy lungs.
Consequently, the resonances of the vocal tract are weaker.
It is both practically and ethically problematic to measure,
with a nasendoscope, the opening of the glottis of a human
yidaki player during performance. For this and other reasons,
we have made studies using an artificial system for playing
the yidaki, in which the "glottis" opening in the artificial
vocal tract can be accurately controlled. Measurements on
such systems show weaker resonances and weaker formants when
the glottis is open. This is in agreement with simple mathematical
models.
Vocalisation while playing
Another feature of yidaki playing is the use of "circular breathing".
In the sound file below, the player (LH)
begins with a steady, sustained note -- without vocalisation.
This is followed by a series of segments in which the drone
is played with the lips while the player vocalises (sings, or
allows his vocal folds to vibrate) at a range of pitches. The
third sound segment in this sound file is analysed in Figure
11 of the experimental
paper. One can hear the vocalisation at a musical fifth
above the drone, so its frequency is 3/2 times that of the drone.
One can also hear a strong harmonic partial a musical seventeenth
above the drone, because the fifth harmonic lies in the formant
of the radiated sound: it is strongly emphasised by the tract
resonance near that frequency.
. . . Download sound file in .wav format (400 k)
The yidaki project combines several different elements. On the
experimental side, the major advance has been the development
of a system that can measure the acoustical impedance of the
vocal tract during performance on the yidaki. In this situation,
the sound level in the mouth can be 100 dBA, which seriously
complicates the making of mesaurements. Other aspects of the
project involve theoretical modelling of the instrument, the
lips, the vocal tract and the lower airway and their interaction.
Much of this work is applicable to other musical instrument-player
interactions, and indeed to other aspects of acoustics.
Several people have worked or are working on various aspects
of the yidaki project:
Alex
Tarnopolsky [1], Neville
Fletcher [1,2], Benjamin
Lange [1], Lloyd
Hollenberg [3], John
Smith [1] and Joe
Wolfe [1].
[1] The University of
New South Wales, [2] The
Australian National University and [3] The
University of Melbourne. |